Preparation and characterization of HM-Lipo-ICG
Lipo-ICG was prepared using a thin-layer hydration method [22, 40]. Cell membranes from B16F10 and G422 GBM cells were isolated and integrated into Lipo-ICG through continuous physical extrusion, resulting in the formation of HM-Lipo-ICG [24, 41]. Transmission electron microscopy (TEM) images revealed uniform spherical shapes with nanoshells of a protein corona in HM-Lipo-ICG, contrasting with Lipo-ICG, confirming successful membrane integration (Fig. 1A, B). Dynamic light scattering (DLS) measurements indicated a smaller hydrated dynamic size for HM-Lipo-ICG (98.8 ± 0.8 nm, PDI = 0.145) compared to Lipo-ICG (117.6 ± 4.6 nm, PDI = 0.191), attributed to physical compression during membrane modification (Fig. 1C, Fig. S1). HM-Lipo-ICG maintained stable hydrodynamic size in water, phosphate-buffered solution (PBS), and 10% fetal bovine serum (FBS) over 10 days, demonstrating excellent stability (Fig. S2). The zeta potential of HM-Lipo-ICG (-36.3 ± 1.0 mV) was higher than that of Lipo-ICG (-45.6 ± 0.4 mV) (Fig. S3), indicating surface potential alteration due to membrane incorporation (-37.3 ± 2.9 mV). SDS-polyacrylamide gel electrophoresis (SDS-PAGE) confirmed the presence of primary protein bands from B16F10 and G422 cell membranes in the HM-Lipo-ICG band (Fig. 1D), verifying successful membrane fusion. Furthermore, dual-channel fluorescence microscopy of FITC and Cy5 dye-labeled membranes showed high overlap in HM-Lipo-ICG aggregates (Fig. 1E), confirming successful co-modification. The encapsulation efficiency and the loading capacity of ICG in HM-Lipo-ICG were calculated to be 77.6 ± 3.7% and 3.7% ± 0.2%, respectively.
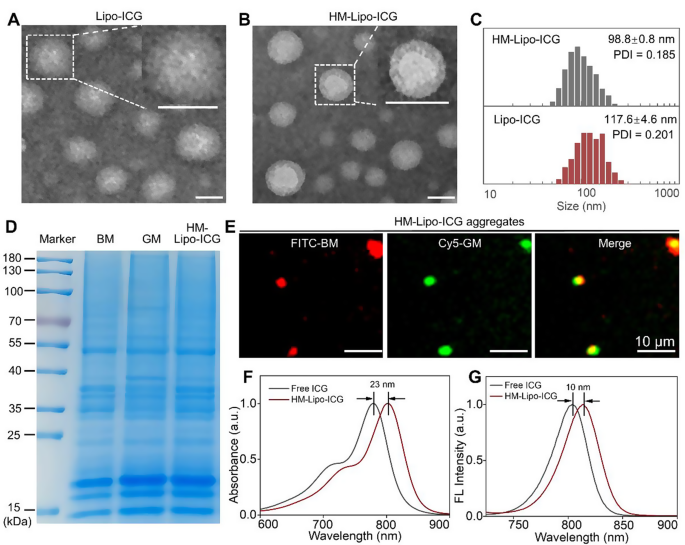
Characterization of HM-Lipo-ICG. a) TEM images of Lipo-ICG and b) HM-Lipo-ICG. Samples were negatively stained with phosphotungstic acid. Insets display a magnified view of a single liposome. Scale bar = 100 nm. c) Hydrodynamic diameter of Lipo-ICG and HM-Lipo-ICG measured by DLS. d) SDS-PAGE protein analysis of marker, B16F10 cell membrane (BM), G422 cell membrane (GM), and HM-Lipo-ICG. e) Fluorescence microscopy images of HM-Lipo-ICG aggregates with FITC-labelled B16F10 cell membrane (red) and Cy5-labelled G422 cell membrane (green). Scale bar = 10 μm. HM-Lipo-ICG with 50 µL at a concentration of 0.1 mg/mL was added dropwise to slides and allowed to dry at room temperature under light-protected conditions. f) Normalized absorption spectra and g) fluorescence spectra of free ICG and HM-Lipo-ICG. CICG = CHM−Lipo−ICG = 20 µg/mL
The photophysical properties of HM-Lipo-ICG were investigated. UV-vis-NIR absorption and fluorescence spectra were similar to free ICG (Fig. 1F, G), indicating no chemical alteration due to the hybrid membrane coating strategy [19]. However, an absorption peak redshift from 780 nm to 803 nm (Fig. 1F) and a fluorescence intensity redshift from 804 nm to 814 nm (Fig. 1G) was observed, attributed to free ICG encapsulation in the liposome’s hydrophilic core.
Optical stability assessment showed a significant improvement in HM-Lipo-ICG, with only a 20% reduction in fluorescence intensity compared to a 65% reduction in free ICG after 6 days of storage (Fig. S4), highlighting enhanced stability conferred by liposomal encapsulation.
In Vitro targeted BBB penetration of HM-Lipo-ICG
The CD44 protein on the surface of B16F10 cells [42, 43] can specifically interact with the hyaluronic acid (HA) on the surface of bEnd.3 cells [36, 44]. Therefore, the CD44 marker on the HM-Lipo-ICG was further detected by western-blotting(WB) measurements. The expression of CD44 in both the BM-Lipo-ICG and HM-Lipo-ICG (Fig. S5) was detected, suggesting that the HM-Lipo-ICG inheritated the marker proteins from source cells.
We hypothesized that Lipo-ICG modified with B16F10 cell membranes would enhance cellular uptake, thereby promoting HM-Lipo-ICG’s ability to cross the BBB via receptor-mediated endocytosis [44]. To investigate this, we first assessed the uptake of HM-Lipo-ICG by bEnd.3 cells using confocal fluorescence imaging and flow cytometry. Results indicated a higher ICG fluorescence signal in the HM-Lipo-ICG-treated group compared to the Lipo-ICG-treated group. Conversely, in the CD44 antibody-blocked group, the ICG signal in bEnd.3 cells was significantly reduced compared to the HM-Lipo-ICG-treated group (Fig. 2A). Flow cytometry quantitative analysis revealed that the mean fluorescence intensity of the HM-Lipo-ICG-treated group was 1.5 times higher than that of the Lipo-ICG-treated group and 1.4 times higher than that of the CD44 antibody-blocked group (Fig. 2B, C). This result indicates that fusion cell membrane modification enhances the uptake efficiency of Lipo-ICG by bEnd.3 cells, and this enhancement is closely related to the specific interaction between the HA on bEnd.3 cells and CD44 on B16F10 cell membranes.
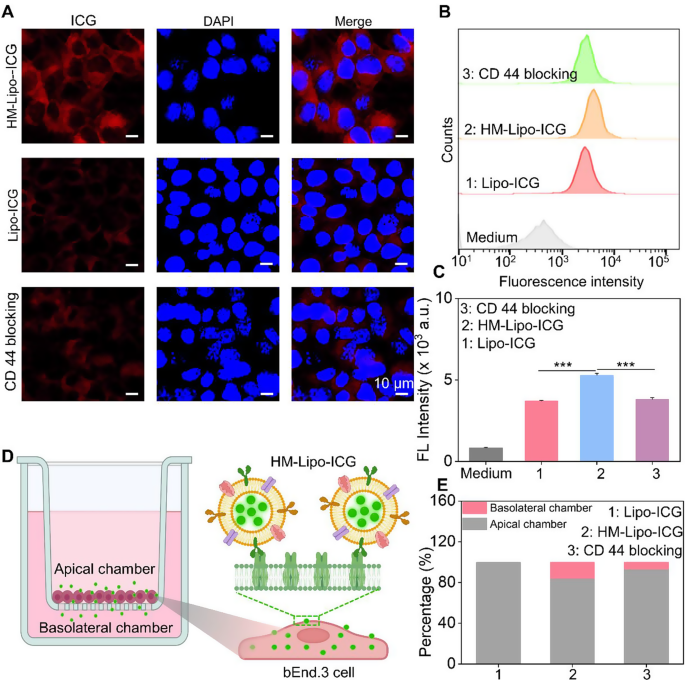
In vitro evaluation of HM-Lipo-ICG for crossing the BBB. a) CLSM images of bEnd.3 cells incubated for 4 h with Lipo-ICG, HM-Lipo-ICG NPs, and HM-Lipo-ICG NPs pre-blocked with CD44 antibody. CICG = CLipo−ICG = CHM−Lipo−ICG = 20 µg/mL. The cell nuclei were stained by DAPI. Scale bar = 10 μm. b) Flow cytometry quantification of bEnd.3 cell uptake of Lipo-ICG, HM-Lipo-ICG, and HM-Lipo-ICG following CD44 antibody blocking. c) Quantitative analysis of the average fluorescent intensity of ICG in Fig. 2B. Data denote mean ± s.d, ∗∗∗ P < 0.001, n = 3. d) Schematic of the in vitro Transwell model. The inset illustrates the mechanism by which HM-Lipo-ICG crosses the BBB through receptor-mediated endocytosis. e) Quantitative analysis of the ability of Lipo-ICG, HM-Lipo-ICG and HM-Lipo-ICG pre-blocked with CD44 antibody to traverse the in vitro BBB model (n = 3). The amount of ICG in the upper and lower chambers was measured and calculated using a linear standard curve
Next, an in vitro BBB Transwell model [44] was established using bEnd.3 cells to preliminarily evaluate the ability of HM-Lipo-ICG to traverse the BBB (Fig. 2D). The trans-endothelial electrical resistance (TEER) of the BBB Transwell model was measured to confirm model reliability (data not shown). Results showed that the relative proportion of ICG in the apical chamber of the HM-Lipo-ICG-treated group (80.8%) was lower than that of the Lipo-ICG (100%) and CD44 blocking groups (91.2%) (Fig. 2E). Conversely, the proportion of ICG in the basolateral chamber of the HM-Lipo-ICG-treated group (19.2%) was higher than that of the Lipo-ICG (0%) and CD44 blocking groups (8.8%) (Fig. 2E). This confirms that fusion cell membrane significantly enhances the ability of Lipo-ICG to traverse the in vitro BBB.
Homotypic targeting of HM-Lipo-ICG to glioma cells in Vitro
Before assessing the homologous targeting performance of HM-Lipo-ICG, its cytotoxicity to G422 GBM cells and bEnd.3 cells was evaluated using the Cell Counting Kit-8 (CCK-8) assay (Fig. S6). The results demonstrated that HM-Lipo-ICG exhibits high cell viability even at an ICG concentration of 40 µg/mL, which is eight times higher than the concentration used for in vivo imaging [45]. This finding underscores the favorable cellular biocompatibility of HM-Lipo-ICG, confirming its suitability for safe use in both tumor imaging and therapeutic applications.
Next, the homologous targeting efficacy of HM-Lipo-ICG to G422 GBM cells was investigated using confocal laser scanning microscopy (CLSM) and flow cytometry. HM-Lipo-ICG, Lipo-ICG, BM-Lipo-ICG, and GM-Lipo-ICG were incubated with G422 cells for 2 h, followed by fixation and DAPI staining (Fig. 3A). The results showed a distinct fluorescent signal from ICG (shown in red) in the HM-Lipo-ICG-treated and GM-Lipo-ICG-treated groups, indicating a significant increase in cellular uptake by G422 cells (Fig. 3B). This observation underscores the commendable self-recognition capacity of HM-Lipo-ICG and GM-Lipo-ICG towards homologous cells, a crucial aspect for achieving targeted cancer cell capabilities [46, 47]. Quantitative analysis by flow cytometry revealed that the average fluorescence intensity in HM-Lipo-ICG and GM-Lipo-ICG-treated cells exceeded that in Lipo-ICG-treated G422 cells by a factor of 1.5 (Fig. 3C and D). This further substantiates that HM-Lipo-ICG and GM-Lipo-ICG, by inheriting cell membrane proteins from G422 cells, efficiently enter glioma sites through homologous targeting.
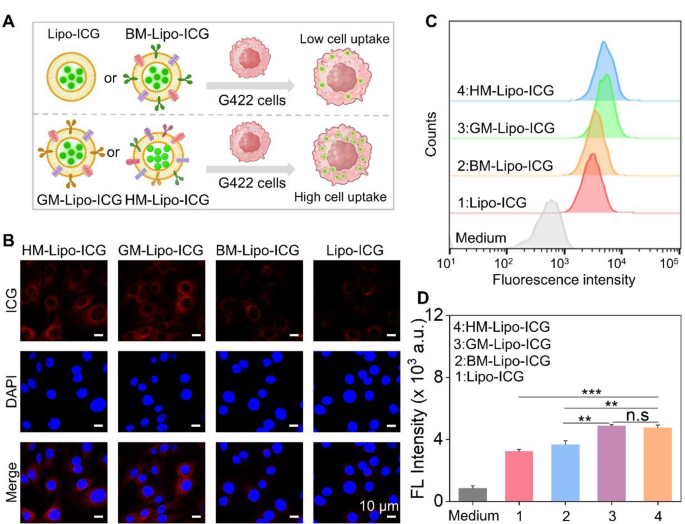
Uptake of HM-Lipo-ICG by G422 glioma cells. a) Schematic of G422 glioma cell uptake of Lipo-ICG, BM-Lipo-ICG, GM-Lipo-ICG, and HM-Lipo-ICG. Image was created with BioRender.com, with permission.b) CLSM images of G422 glioma cells incubated with Lipo-ICG, BM-Lipo-ICG, GM-Lipo-ICG, and HM-Lipo-ICG for 2 h. Cell nuclei were stained with DAPI. CICG = 20 µg/mL. Scale bar = 10 μm. c) Flow cytometry analysis of G422 cell uptake of Lipo-ICG, BM-Lipo-ICG, GM-Lipo-ICG, and HM-Lipo-ICG. d) Mean fluorescence intensity of ICG in G422 glioma cells treated with Lipo-ICG, BM-Lipo-ICG, GM-Lipo-ICG, and HM-Lipo-ICG for 2 h (n = 3) in Fig. 3C. ns, ∗, ∗∗, and ∗∗∗ represent no statistical difference, P < 0.05, P < 0.01, and P < 0.001, respectively
Additionally, to comprehensively evaluate the performance of HM-Lipo-ICG in a realistic GBM brain condition, an in vitro BBB model was established by seeding endothelial cells in the upper chamber and GBM cells in the lower chamber (Fig. S7A). Subsequently, HM-Lipo-ICG, GM-Lipo-ICG, and Lipo-ICG were separately introduced into the upper chamber to assess their ability to penetrate the BBB and be taken up by glioma cells using confocal laser scanning microscopy (CLSM). The results obtained from CLSM imaging (Fig. S7B) and quantitative analysis (Fig. S7C) demonstrated that the group treated with HM-Lipo-ICG exhibited superior BBB penetration and active-targeting capabilities compared to both the GM-Lipo-ICG-treated group and Lipo-ICG-treated group. These findings highlight that through modification with homologous cell membrane, hybrid cell membrane camouflaged Lipo-ICG demonstrates excellent tumor targeting abilities after crossing the BBB (Fig. S7).
Finally, to assess whether the coating of tumor cell membranes protects against phagocytosis by macrophages, the cellular uptake of HM-Lipo-ICG by RAW264.7 cells was investigated using CLSM and flow cytometry. The results revealed that Lipo-ICG-treated cells exhibited increased red luminescence, whereas HM-Lipo-ICG-incubated cells showed a 1.5-fold reduction in intracellular fluorescence (Fig. S8). This suggests that only a minimal number of cell membrane-coated liposomes are internalized by RAW264.7 macrophages, underscoring the enhanced phagocytosis-evading capabilities conferred by the biofunctional properties inherited from the source cancer cells [46, 48].
In Vivo targeted BBB penetration of HM-Lipo-ICG
Encouraged by the successful traversal of the in vitro Transwell BBB by HM-Lipo-ICG, we further evaluated the ability of HM-Lipo-ICG to penetrate the BBB and enter brain tissue in a normal mouse model using NIR fluorescence imaging. Four different liposomal formulations, including HM-Lipo-ICG, Lipo-ICG, GM-Lipo-ICG, and BM-Lipo-ICG, were administered via intravenous injection (1.0 mg/kg per mouse), and real-time NIR fluorescence imaging was performed at various time points. As illustrated in Fig. 4A, the groups injected with Lipo-ICG and GM-Lipo-ICG exhibited minimal fluorescence signals in the brain regions of healthy mice. In stark contrast, the groups receiving BM-Lipo-ICG and HM-Lipo-ICG showed significantly higher fluorescent signals, confirming that the use of metastatic cancer cell membrane camouflage conferred exceptional BBB permeability to the liposomes, allowing them to penetrate the brain even when the BBB was intact. Quantitative analysis revealed that, at 6 h post-injection, the fluorescence intensity in the brains of mice treated with HM-Lipo-ICG and BM-Lipo-ICG was approximately 2.8 times higher compared to those treated with Lipo-ICG and GM-Lipo-ICG (Fig. 4B). No statistically significant difference in fluorescence intensity was observed between the Lipo-ICG and GM-Lipo-ICG groups, nor between the BM-Lipo-ICG and HM-Lipo-ICG groups. These findings suggest that cell membrane-coated biomimetic liposomes derived from brain metastatic cancer cells retain the functional properties of the original cell membrane, thus enhancing their BBB penetration capabilities. Additionally, ex vivo imaging of dissected brains corroborated the in vivo results, showing substantial fluorescent signals in the BM-Lipo-ICG and HM-Lipo-ICG groups (Fig. 4C).
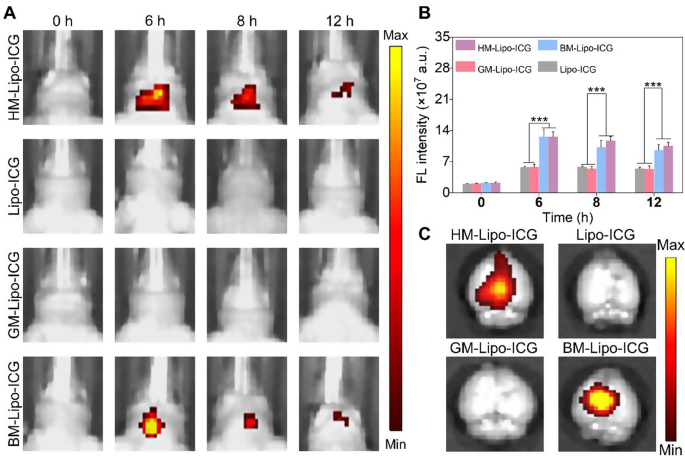
HM-Lipo-ICG crossing the BBB in normal mice. a) NIR fluorescence imaging of normal mouse brains at various time points following intravenous injection of HM-Lipo-ICG at a dose of 1.0 mg/kg. b) Quantitative analysis of fluorescence intensity in mouse brains from Fig. 4A. n = 3. c) Ex vivo NIR fluorescence images of brain tissue from different treatment groups 6 h post-intravenous injection
In vivo imaging of infiltrative tumor margins
The G422 orthotopic glioma mouse model was established using methods previously described by our group [22]. This model was utilized to evaluate the in vivo and microscopic near-infrared (NIR) fluorescence imaging capabilities of HM-Lipo-ICG for visualizing tumor margins (Fig. 5A). Luciferase-stably transfected G422 cells exhibited strong bioluminescent signals in the mouse brain 9 days post-establishment (Fig. 5B and Fig S9). Gadolinium-based T1-weighted contrast-enhanced magnetic resonance imaging also delineated the brain tumor region in vivo (Fig. 5C). Furthermore, histopathological analysis of whole brain tissue from model mice using hematoxylin and eosin (H&E) staining revealed areas of infiltrative tumor margins within the brain tissue (Fig. 5D). These findings collectively confirm the successful establishment of an infiltrative GBM orthotopic mouse model.
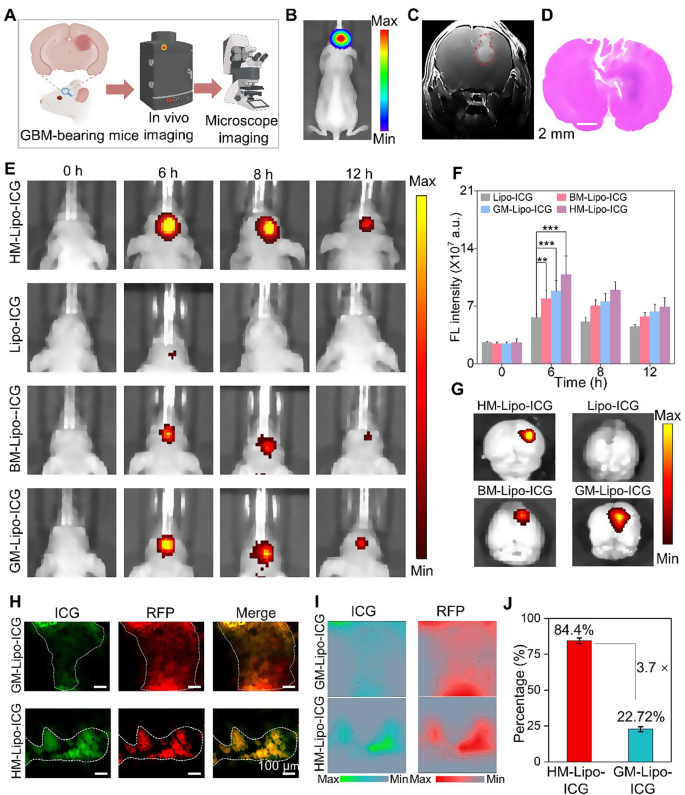
HM-Lipo-ICG for accurate visualization of infiltrative tumor margins in an orthotopic glioma mouse model. a) Schematic of the G422 orthotopic glioma model construction and its application in fluorescence and microscopic NIR fluorescence imaging in vivo. b) A bioluminescence image and c) a magnetic resonance image of a G422 orthotopic glioma mouse. d) H&E-stained histological section of brain tissue of G422 glioma cells in mouse brains 9 days post-implantation. Scale bar = 10 μm. e) NIR fluorescence images of G422 tumor-bearing mice treated with Lipo-ICG, BM-Lipo-ICG, GM-Lipo-ICG, and HM-Lipo-ICG at various time points. Intravenous dose of ICG = 1.0 mg/kg. f) Quantitative analysis of fluorescence signal intensity in the mouse brain regions in Fig. 5E. g) Ex vivo fluorescence images of brain tissue 4 h post-injection of Lipo-ICG, BM-Lipo-ICG, GM-Lipo-ICG, and HM-Lipo-ICG. h) NIR fluorescence microscopy images of brain tumor Sect. 6 h post-injection of GM-Lipo-ICG and HM-Lipo-ICG, respectively. Scale bar = 10 μm. i) Quantitative fluorescence intensity distribution map of Fig. 5H. The map is obtained by extracting and normalizing the fluorescence intensity signals from Fig. 5D and projecting them onto the corresponding positions. Scale bar = 10 μm. j) Quantitative analysis of the results from Fig. 5I (n = 3)
Subsequently, the in vivo NIR fluorescence imaging capability of HM-Lipo-ICG for visualizing infiltrative tumor margins was assessed using the IVIS imaging system. Following intravenous injection of HM-Lipo-ICG (1.0 mg/kg per mouse), NIR fluorescence imaging was performed at various time points (Fig. 5E). The results demonstrated progressive fluorescence signals within the brain tumor region in the HM-Lipo-ICG, BM-Lipo-ICG, and GM-Lipo-ICG treatment groups, peaking at 6 h post-injection before gradually declining. In contrast, minimal fluorescence was observed in the Lipo-ICG-treated group within the mouse brain. Quantitative analysis indicated that at 6 h post-injection, fluorescence intensity at the tumor sites of BM-Lipo-ICG and HM-Lipo-ICG-treated mice was approximately 1.4-fold and 1.9-fold higher, respectively, compared to Lipo-ICG-treated mice (Fig. 5F). Following Fig. 4A, the peak accumulation of HM-Lipo-ICG in the targeted tumor regions is indicated by the highest fluorescence intensity observed at 6 h post-injection. The decline in fluorescence intensity over time can be attributed to a combination of liposome clearance through lymphatic and circulatory systems, metabolic degradation, and potential cellular uptake. HM-Lipo-ICG enabled high-contrast NIR imaging with a signal-to-background ratio of 6.5 in GBM regions within the orthotopic glioma mouse model. Ex vivo imaging of brain tissues from different treatment groups corroborated the in vivo fluorescence imaging results (Fig. 5G), confirming the superior homotypic targeting ability of G422 glioma cell membrane-camouflaged liposomes. Importantly, fluorescence intensity in gliomas of the HM-Lipo-ICG-treated group was significantly greater than in the GM-Lipo-ICG and BM-Lipo-ICG-treated groups, underscoring that HM-Lipo-ICG nanoparticles effectively traverse the BBB surrounding emerging gliomas and exhibit specific tumor-targeting capabilities.
Additionally, NIR fluorescence microscopic imaging of HM-Lipo-ICG was employed to delineate infiltrative tumor margins in frozen sections of brain tissues. For this purpose, RFP-transfected G422 (RFP-G422) cells were used to establish orthotopic glioma mouse models. As depicted in Fig. 5H, brain tissue sections from the HM-Lipo-ICG-treated group showed complete overlap between red fluorescence emitted by glioma cells and green fluorescence emitted by ICG. In contrast, green fluorescence of ICG in the GM-Lipo-ICG-treated group did not fully correspond with red fluorescence at glioma margins, indicating that liposomes modified with hybrid cell membranes can more accurately identify infiltrative glioma margins compared to those modified with single glioma cell membranes. Further quantitative analysis (Fig. 5I, J) revealed that the agreement between tumor areas identified by the HM-Lipo-ICG-treated group and actual tumor areas was as high as 84.4%, approximately 3.7 times higher than that of the GM-Lipo-ICG-treated group (22.7%).
Finally, the biodistribution and metabolic pathways of HM-Lipo-ICG in normal mice were evaluated. Ex vivo NIR fluorescence imaging (Fig S10) revealed that 6 h post-intravenous injection, HM-Lipo-ICG primarily accumulated in liver tissue, consistent with findings for Lipo-ICG, BM-Lipo-ICG, and GM-Lipo-ICG. However, signal intensity in liver tissue was lower in the HM-Lipo-ICG-treated group compared to the Lipo-ICG-treated group, suggesting that cell membrane modification can reduce reticuloendothelial system (RES) uptake of Lipo-ICG, thereby enhancing imaging quality.
Fluorescence image-guided surgical resection of GMB in vivo
HM-Lipo-ICG demonstrated a remarkable ability to identify infiltrative tumor margins, highlighting its potential for guiding fluorescence imaging-directed surgical resection. To assess its applicability in facilitating precise neurosurgery for GBM, craniotomies were performed with stepwise glioma tissue removal guided by NIR fluorescence imaging of HM-Lipo-ICG, as depicted in Fig. 6A. A representative brain bearing GBM was collected 6 h post-injection of HM-Lipo-ICG. The tumor region, highlighted by a blue circle in Fig. 6C, was exposed and subjected to sequential resections to excise GBM tissue. Before initial resection, the tumor protruded from the brain surface, emitting strong fluorescence signals (Fig. 6B). Following the first resection, the tumor mass visibly disappeared under direct observation, yet significant fluorescence persisted in the resection bed. Subsequently, a second resection removed remaining fluorescent tissue, creating a small cavity on the brain surface. However, a faint area of residual fluorescence was detected after the second resection, indicating a residual tumor in the surgical cavity. Therefore, a third resection was conducted, resulting in complete disappearance of fluorescence. Quantitative analysis revealed that stepwise glioma removal reduced fluorescence at the tumor site from 80.0 (pre-resection) to 27.4 (first resection), 12.0 (second resection), and ultimately to zero (Fig. 6D). To validate surgical outcomes, resected tissue was examined using hematoxylin and eosin (H&E) staining (Fig. 6E). Initial H&E staining of dissected tumor tissue revealed a dense population of tumor cells, gradually diminishing with successive resections. Following disappearance of fluorescence, the resection bed displayed normal brain tissue. These findings emphasize that HM-Lipo-ICG-based NIR fluorescence imaging represents a promising strategy for guiding precise and comprehensive neurosurgery, effectively targeting gliomas and delineating tumor margins.
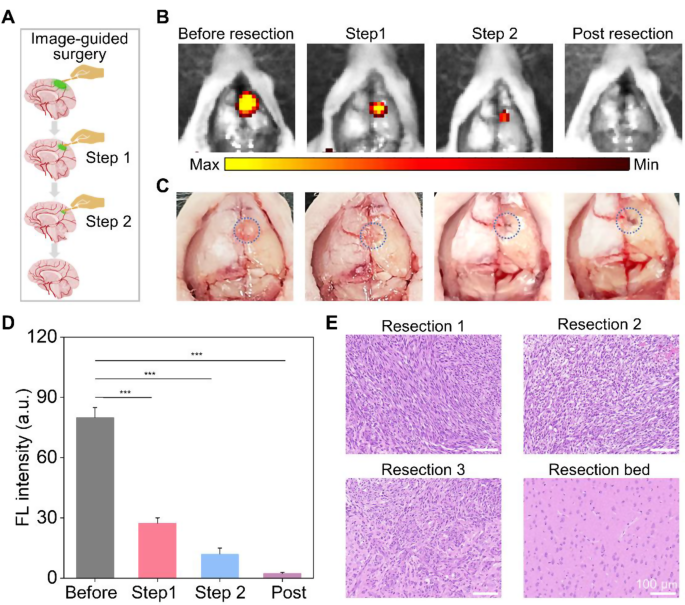
In vivo NIR fluorescence imaging-guided glioma surgery in G422 orthotopic glioma models. a) Schematic representation of the stepwise surgical procedure. b) and c) Digital and NIR fluorescence images of the tumor resection site, taken 6 h post-injection of HM-Lipo-ICG nanoparticles. The blue dashed circle in Fig. 6C delineates the tumor location. The surgery continued until all regions emitting a bright fluorescent signal were completely excised. d) Quantitative analysis of fluorescence signal intensity in the mouse brain regions in Fig. 6B. e) Representative H&E-stained sections of the resected tumor tissues following each surgical stage. Scale bar = 100 μm
In vivo toxicity evaluation
The in vivo cytotoxicity of Lipo-ICG, GM-Lipo-ICG, BM-Lipo-ICG, and HM-Lipo-ICG was rigorously assessed through a comprehensive analysis of blood biochemistry. Mice treated with PBS as the control. Results showed that the mice treated with nanoparticles exhibited negligible changes in three key liver function markers compared to the control group (Fig. S11), including alanine aminotransferase (ALT), aspartate aminotransferase (AST), and alkaline phosphatase (ALP). Additionally, routine blood parameters, including red blood cell (RBC) count, white blood cell (WBC) count, hematocrit (HCT), mean corpuscular volume (MCV), hemoglobin (HGB), and platelet count (PLT), showed no significant differences between groups. These findings underscore the favorable in vivo biosafety of these nanoparticles, without inducing distinct liver or renal toxicity. Histopathological examination from H&E-stained slices of major organs (heart, liver, lung, spleen, and kidney) also revealed no discernible pathological tissue changes or damage in the different treated groups compared with the control group (Fig. S12), indicating the absence of side effects or toxicities induced by these liposomes or cell membrane coating. Taken together, these results confirm that HM-Lipo-ICG exhibits excellent biosafety and that the hybrid tumor cell membrane cloaked strategy did not affect the normal function of the human tissues, holding significant promise for clinical application.